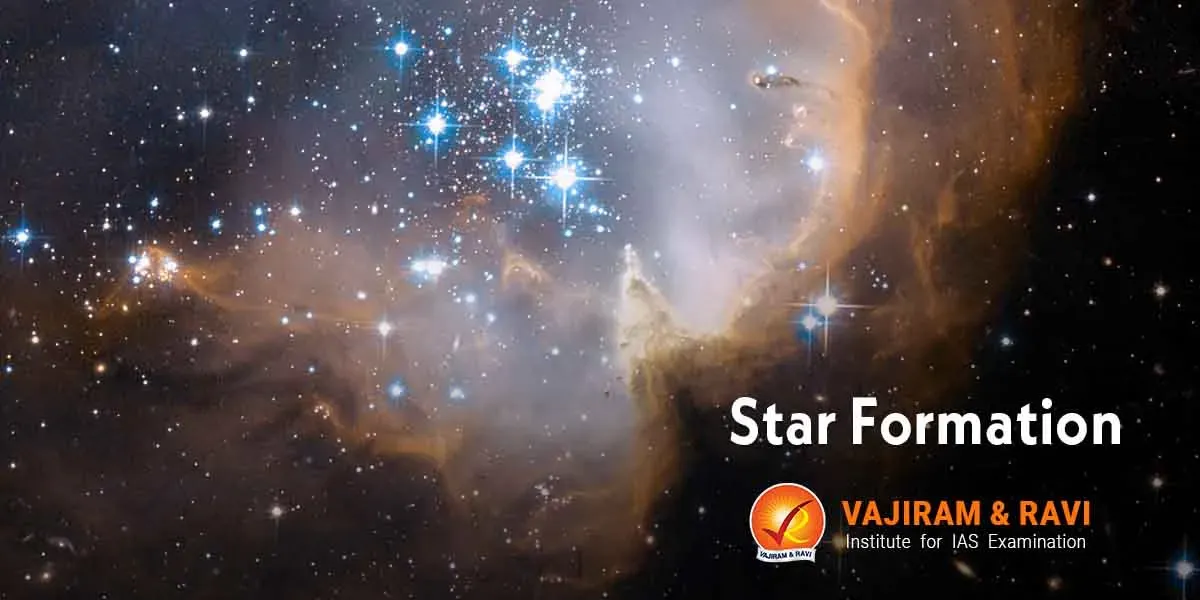
28-03-2024
GS-III
Sub-Categories:
GS-III: Science & Technology
Prelims: General Science
Mains: Science and Technology- developments and their applications and effects in everyday life.
Star formation is the process by which the dense regions within molecular clouds of dust and gases collapse to form stars. This process begins when some of these dense clusters reach a critical density and start to collapse under their own gravity resulting in the formation of a hot core, known as a protostar. This hot core at the heart of the collapsing cloud eventually becomes a Star.
Stars are giant balls of hot gas, mainly hydrogen with some helium and trace amounts of other elements. There are approximately 10^24 stars in the Universe.

What is a Star?
Stars are individual celestial bodies composed mostly of hydrogen and helium that undergo nuclear fusion, emitting light and heat. They are recognised as the fundamental building blocks of galaxies. They are giant, luminous spheres of plasma.
- The age, distribution, and composition of the stars in a galaxy help in tracing the history and evolution of that galaxy.
- There are 200 billion to a few trillion galaxies present in the observable universe.
- The Milky Way contains around 100 billion stars, including our Sun.
- Stars are responsible for the distribution and manufacturing of heavy elements such as carbon, nitrogen, and oxygen.
- The study of stars i.e., their birth, life, and death is central to the field of astronomy.
- Proxima Centauri is the closest star to Earth (other than the Sun) which is a Red dwarf.
Variable Stars
Variable stars are those stars that change their apparent brightness over time. There are two main types of variable stars - intrinsic and extrinsic.
- Intrinsic Variables: These stars change in brightness due to some change within the star itself, such as pulsation or eruption.
- For example, Cepheids are intrinsic variables.
- Extrinsic Variables: The apparent changes in brightness of these stars are due to changes in the amount of their light that can reach Earth.
- For example, an eclipsing binary star dims when it is eclipsed by a companion, and then brightens when the occulting star moves out of the way.
- The Sun belongs to both categories as it is intrinsic because of sunspots and extrinsic because of the eclipse.

Life Cycle of Stars
The stellar evolution infers the process and life cycle of the star from its formation to decay and ultimately death of a star.
Formation of Star
The formation process of stars undergoes multiple stages till it becomes the Main Sequence Star such as the Sun. The stages are as follows:

- Cloud Dust: Stars emerge within vast clouds of dust and gas scattered throughout galaxies, such as the Orion Nebula.
- These regions are also referred to as "stellar nurseries" or "star-forming regions".
- The turbulence within these clouds causes knots with sufficient mass to initiate gravitational collapse. This collapse leads to the formation of a hot core known as a protostar.

- Protostar: The protostar is a dense and hot core at the centre of the collapsing cloud.
- It gathers surrounding dust and gas, eventually becoming the precursor to a fully developed star.
- T Tauri Star: T Tauri stars represent young, pre-main-sequence stars with irregular brightness variations and strong stellar winds. They bridge the gap between protostars and stable main sequence stars.
Main Sequence Stars
Stars spend the majority of their lives in the stable phase known as the main sequence, as depicted on the Hertzsprung-Russell Diagram. Our Sun is currently a main sequence star.

- Equilibrium: The cores of main-sequence stars are in hydrostatic equilibrium, where outward thermal pressure from nuclear reactions at the star's core balances the inward pull of gravity, making them stable.
- It is the longest phase of any star’s life.
- Fusion: This phase involves nuclear fusion in the star's core, converting hydrogen into helium, and provides the energy to support the star against gravitational collapse.
- Once hydrogen burning in the core ceases, the star loses this stability and evolves away from, or off, the main sequence, becoming a red giant or supergiant.
- Lifespan: The lifespan of a main sequence star depends on its mass.
- More massive stars burn through their fuel faster and have shorter lifespans.
- Low-mass stars, such as red dwarfs will shine for tens of billions of years whereas; massive stars live just for a few million years.
- Red Dwarfs: Main Sequence stars exhibit diverse luminosities and colours. Red dwarfs are the smallest stars, constituting up to 10% of the Sun's mass, emitting minimal energy and glowing feebly at temperatures between 3000-4000K.
- Despite their small size, red dwarfs are abundant and endure for tens of billions of years.
Death of Star
When a star exhausts the hydrogen in its core, it undergoes changes leading to its eventual fate.
- Red Giant and Super Giant: When a main sequence star, less than eight times the Sun's mass, exhausts its core hydrogen, it begins to collapse.
- The increasing temperature and pressure cause helium to fuse into carbon in the core.
- The hydrogen fusion moves into outer layers, leading to the expansion of the outer layers, forming a red giant with an orange hue.
- In approximately 5 billion years, the Sun will evolve into a red giant.

- Planetary Nebula: The red giant becomes unstable and begins pulsating, periodically expanding and ejecting some of its atmosphere.
- Eventually, all of its outer layers blow away, creating an expanding cloud of dust and gas called a planetary nebula.

- White Dwarfs: Average stars like our Sun near the end of their life cycle shed outer layers exposing only the stellar core, resulting in a White Dwarf.
- Despite being a stellar remnant, White Dwarfs retain intense heat, resembling glowing cinders.
- They remain stable with the pressure from fast-moving electrons preventing complete gravitational collapse.
- The more massive the core, the denser the resulting white dwarf will be.

- Chandrasekhar Limit: The fate of becoming white dwarfs is available to only those stars which have a mass of up to1.4 solar mass (called Chandrasekhar Limit, named after S. Chandrashekhar).
- Stars within this limit (white dwarfs) may undergo nova explosions.
- Stars heavier than this limit undergo supernovas leaving behind neutron stars or black holes.
- Nova Explosion: If a white dwarf has any companion nearby (in binary or multiple star systems), it may undergo a nova.
- It occurs when the gravity of the white dwarfs drags matter (mainly hydrogen) from the companion star (s) resulting in brightening.
- This brightening (explosion) is due to nuclear fusion after enough hydrogen accumulation and expulsion of the remaining matter.
- Sometimes, massive white dwarfs (near the 1.4 solar mass limit) may explode completely, becoming a supernova.

- Supernova Explosion: Massive stars with more than eight solar masses are destined to undergo supernovae.
- In these massive stars, a complex series of fusion takes place - helium to carbon, carbon to heavier elements, and ultimately silicon to iron.
- Now fusion is no longer supported, the iron core first collapses then rebounds - creating a shock wave, and then a huge explosion called a supernova occurs.
- Supernovae leave behind remnants such as neutron stars or black holes.

- Neutron Stars: If the collapsing core of the supernova has 1.4 to 3 solar masses, it further collapses until the formation of neutrons by the combination of electrons and protons, thereby producing a neutron star.
- They are extremely dense (similar to atomic nuclei) due to immense gravity.
- Due to accreting gas from nearby companions, in a multiple-star system, neutron stars emit X-rays.
- They have powerful magnetic fields that create beams of radiation.
- Pulsars: The magnetic field of a rotating neutron star can be observed as pulses, if Earth is in their path, classifying them as pulsars.
- Magnetars: All neutron stars have strong magnetic fields. But a magnetar can be 10 trillion times stronger than a refrigerator magnet and up to a thousand times stronger than a typical neutron star’s.

- Black Holes: When a stellar core collapses beyond three solar masses, it undergoes a complete transformation into a black hole which is an infinitely dense entity with gravity so intense that not even light can escape its immediate vicinity.
- Detection of black holes relies on indirect observations due to their elusive nature.
- The gravitational field of a black hole captures nearby material, from the outer layers of a companion star, forming a heated disk (Accretion disks).
- This process emits substantial X-rays and Gamma-rays, providing observable crucial cosmic signatures.
- Black holes, with their inscrutable gravitational influence, stand as cosmic phenomena reshaping our understanding of the universe.
The dust and debris left behind by novae and supernovae eventually blend with the surrounding interstellar gas and dust, eventually recycled and providing the building blocks for a new generation of stars.
PYQs on Star Formation
Question 1: Consider the following pairs: (UPSC 2023)
Objects in space: Description
- Cepheids: Giant clouds of dust and gas in space
- Nebulae: Stars which brighten and dim periodically
- Pulsars: Neutron stars that are formed when massive stars run out of fuel and collapse
How many of the above pairs are correctly matched?
- Only one
- Only two
- All three
- None
Answer: (a)
FAQs on Star Formation
Where does star formation occur?
Star formation occurs predominantly within large molecular clouds, often located in the spiral arms of galaxies. These clouds are rich in molecular hydrogen and other compounds, providing the necessary raw materials for the birth of stars.
How do stars form from molecular clouds?
The process begins with a molecular cloud fragmentation under its own gravity. As the cloud contracts, it forms a rotating disk and a central protostar. Material from the disk accretes onto the protostar, leading to increased temperature and pressure until nuclear fusion begins, marking the birth of a star.
How long does star formation take?
The timescale for star formation varies, but it typically ranges from tens of thousands to millions of years. Factors such as the mass of the forming star and the surrounding environment influence the duration of this process.
What is a protostar?
A protostar is a young stellar object that forms from the gravitational collapse of a region within a molecular cloud. It represents an early stage in the star formation process, where the central core has not yet initiated sustained nuclear fusion.
What types of stars result from the formation process?
The mass of the collapsing molecular cloud determines the type of star that forms. High-mass clouds can lead to the formation of massive, short-lived stars, while low-mass clouds may give rise to smaller, longer-lived stars like our Sun.