A tokamak is a device designed to harness nuclear fusion, the reaction that powers the sun and all the stars. In a tokamak, magnetic field coils confine plasma to achieve the required conditions for fusion on the Earth. The first tokamak, T-1 began operation in Russia in 1958 and the subsequent advances led to the construction of the Tokamak Fusion Test Reactor at Princeton Plasma Physics Laboratory and the Joint European Torus in England, both of which achieved record fusion power in the 1990s.
Tokamak's sophisticated technology aims to provide an abundant, climate-friendly energy source, which is why 35 nations have collaborated on the superconducting ITER project.
What is Nuclear Fusion?
Nuclear fusion is a process whereby light nuclei fuse together to form heavier nuclei and release enormous amounts of energy, much more than is released by nuclear fission. Fusion can be controlled, as occurs in the Sun (and other stars) and fusion reactors or it can be uncontrolled, as in the thermonuclear bombs.
- Thermonuclear fusion: Nuclear fusion is the result of very high-speed collisions of light atomic nuclei, which need extremely high temperatures in a confined space; hence, it is also called thermonuclear fusion.
- Example: Fusion of Deuterium and Tritium (isotopes of hydrogen)
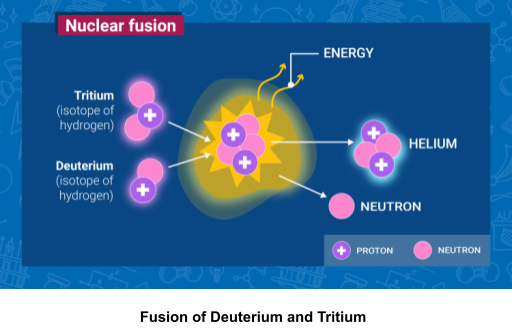
- Nuclear fusion occurring in stars: To fuse in the Sun and other stars, nuclei must collide with each other at very high temperatures, close to ten million degrees C.
- Temperature: This high temperature facilitates enough energy to overcome the electrical repulsion between the like charges of the atoms and nuclei.
- Confinement: In the sun (or other stars), its enormous gravity creates extreme pressure to confine the nuclei in a small space, increasing the chances of collision between nuclei.
- Within this very close range, the nuclear force between the nucleons outweighs the repulsion and allows nuclear fusion.
Artificial Nuclear Fusion
Ever since the discovery of nuclear fusion in the 1930s, harnessing its energy has been a problem for scientists due to the requirement of high temperatures and pressure for the reaction. Thermonuclear bombs (hydrogen bombs) use a small fission bomb to meet the requirements.
Challenges in Harnessing Fusion Energy
To recreate fusion and harness this limitless, clean, and safe energy by using reactors (controlled fusion) has not been easy. Several approaches, such as Tokamak and other devices, have tried to achieve this controlled thermonuclear fusion.
- Extremely high temperatures are needed:
- Fusion of Deuterium and Tritium, the most basic and least difficult to create, and which most of the fusion reactors use, needs 100 million degrees Celsius to occur.
- Fusion takes place in plasma (a state of matter), which is a hot and charged gas made up of positive charges and freely moving electrons, due to this extreme temperature. The confinement of this plasma is very difficult to achieve.
- Maintaining the plasma at 100-150 million°C long enough to sustain fusion requires tremendous heating systems and thermal isolation.
- Plasma instability:
- The charged plasma has inherent instabilities that can cause turbulence and leakage.
- Magnetic confinement using Tokamak and Stellarator devices is currently the most advanced method for harnessing fusion energy.
- However, this confinement must be precisely tuned to keep the plasma dense and stable for a longer period.
Due to these challenges, no fusion plant has yet produced a net energy gain. However, the promise of abundant clean power continues to drive cutting-edge research to make tokamak fusion commercially feasible during this century.
What is a Tokamak Device?
Tokamak, a Russian acronym for a toroidal (torus-shaped) vacuum chamber with magnetic coils, is a complex magnetic confinement device designed to achieve nuclear fusion energy under controlled conditions.
Tokamak Basic Principles
The tokamak enables fusion by creating an extremely hot plasma and confining it tightly using magnetic fields. Here are some key principles:

- Plasma - The starting point is gas, which gets ionized into plasma, comprising freely moving electrons and ions. This plasma flow is channeled into a toroidal chamber.
- Magnetic fields - Powerful magnets create two main magnetic fields: a toroidal field going around the torus and a poloidal field crossing it. This creates a helical field to contain the plasma.
- Confinement - The charged particles spiral along the magnetic field lines without hitting the walls, as fields exert radial inward forces to balance the outward plasma pressure. This traps the plasma in a stable vortex.
- Current drive - Electric currents flowing in the plasma strengthen the confining magnetic fields. External systems drive and sustain these tokamak currents.
- Heating systems - Various electromagnetic waves and particle beams are aimed at the plasma to heat it to over 150 million °C for fusion reactions.
- Fusion- Hydrogen isotopes undergo fusion at such extreme temperatures, releasing energy.
The tokamak thus creates a doughnut-shaped magnetic "bottle" using fields to confine the charged plasma particles so they can fuse and yield energy when heated to millions of degrees. The complex physics enables stable confinement close to ideal conditions.
How is Tokamak different from other devices?
The tokamak differs from other fusion devices in the following key ways:
- Principle: Tokamak uses magnetic confinement to contain the plasma. Other devices may use inertial confinement (lasers/beams heating fuel pellets) or electrostatic confinement (electric fields).
- Shape: Tokamak has a toroidal (doughnut) shaped chamber. Others may be cylindrical (Z-pinch) or pellet-shaped (inertial fusion).
- Confinement Method: Magnetic fields confine the plasma in a torus in tokamaks. Stellarators use twisted three-dimensional magnetic fields.
- Stellarators have advantages like lower power requirements, greater design flexibility, and simplified plasma control compared to tokamaks, but their complex twisted coil geometry poses engineering challenges.
- Z-pinch uses axial current to compress plasma cylindrically.
- Inertial fusion uses rapid implosion.
- Temperature: Tokamaks aim for 10-15 keV (100-150 million°C) plasma. Inertial fusion requires billions of degrees.
- Control: Tokamaks allow fine control over plasma shape, position, and stability parameters. This enables excellent confinement close to ideal fusion conditions.
Significance as a Future Energy Source
Fusion power plants based on the tokamak device hold tremendous promise as future energy sources due to benefits like:
- Abundant fuel source:
- Deuterium can be distilled from seawater, while tritium can be produced from lithium.
- Since the oceans contain enough deuterium to power fusion for billions of years, the fuel supply is, for all practical purposes, unlimited. This ensures energy security.
- No GHG emissions:
- Fusion generates no carbon dioxide or air pollutants during operation.
- The fuels are non-radioactive, and the products are helium gas and neutrons.
- This makes fusion an inherently clean, sustainable energy source that does not contribute to climate change.
- Minimal radioactive waste:
- Tokamak components become radioactive during operation from neutron bombardment. But this radioactivity fades within 50-100 years, rather than millennia.
- So, waste disposal is less hazardous than fission reactors. Furthermore, fusion fuels are only activated while inside the reactor.
India's Tokamak Initiatives
India is actively involved in tokamak research, both participating in the ITER project and developing indigenous capabilities. Key efforts include:
- The Institute for Plasma Research (IPR) in Gandhinagar runs several operational tokamaks like Aditya and SST-1 for fusion research.
- ADITYA-U: ADITYA Upgrade (ADITYA-U) is an upgraded version of ADITYA tokamak, which operated since 1989.
- It is a medium-sized tokamak with a maximum toroidal magnetic field of 1.5 T.
- It has been designed to produce circular plasmas with a current of nearly 150–250 kA and a plasma duration of around 250–350 milliseconds.
- Steady State Tokamak (SST) - 1:
- This medium-sized tokamak with a toroidal magnetic field of 1.5T produces a plasma current of around 100 kA and confines the plasma for nearly 450 milliseconds.
- SST-2: IPR is constructing the superconducting SST-2 Tokamak to handle over 1000 plasma pulses and currents exceeding 1 million amperes.
- This will study stability at high currents.
- SST-3: The Institute is developing a new Steady-State Superconducting Tokamak (SST-3) that will integrate features from SST-2 and ITER using high-temperature superconductors.
- Most ambitiously, IPR is planning the IN-SPARC demonstration reactor to achieve net energy gain from fusion by 2030. This will employ advanced indigenous technologies.
Nuclear Fusion Projects around the World
Some leading experimental fusion reactors and projects around the world include:
- Joint European Torus (JET) - EU project in the UK to study plasmas and fusion technology. Largest operational tokamak today and holds a fusion power record.
- ITER - International nuclear fusion megaproject building the world's largest experimental tokamak in France. The aim is to demonstrate the feasibility of fusion energy.
- National Ignition Facility (NIF) - Inertial confinement facility in the USA focusing on nuclear fusion via laser ignition of fuel pellets.
- Wendelstein 7-X (W7X) - Advanced stellarator in Germany to test magnetic plasma confinement for long durations.
- Large Helical Device (LHD) - Magnetic fusion device in Japan to research helical systems for plasma confinement.
- Korea Superconducting Tokamak Advanced Research (KSTAR) - South Korean fusion facility running high-performance plasmas.
- Experimental Advanced Superconducting Tokamak (EAST) - Chinese magnetic confinement device holding long pulse length records.
- HL-2M Tokamak - China's largest nuclear fusion machine aiming to study confinement physics and engineering.
With growing energy needs and climate concerns, harnessing fusion could be a long-term sustainable energy solution. These cutting-edge facilities aim to turn this dream into reality by advancing fusion science and technology.